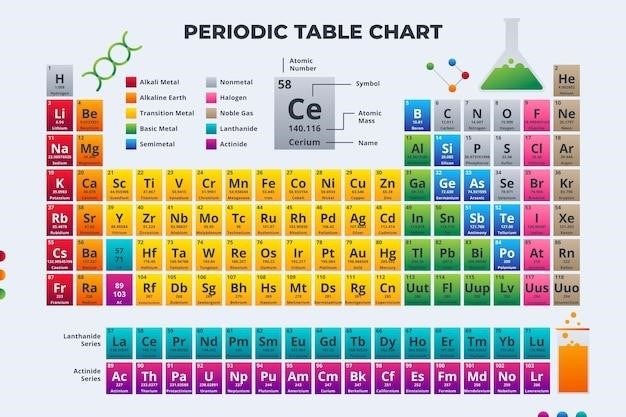
Periodic Table with Electron Configurations⁚ A Comprehensive Guide
This guide explores the relationship between the periodic table and electron configurations. Learn how to predict and understand electron arrangements using the table’s structure. Discover printable periodic tables with electron configurations in PDF format and other resources to aid your understanding. Master the skill of identifying elements from their configurations and explore practical applications in chemistry.
Understanding Electron Configurations
Electron configuration describes the arrangement of electrons within an atom’s orbitals. These orbitals, designated by principal quantum numbers (n), represent energy levels, with lower n values indicating lower energy; Within each energy level are sublevels (s, p, d, f), each capable of holding a specific number of electrons. The s sublevel holds a maximum of two electrons, p holds six, d holds ten, and f holds fourteen. The Aufbau principle dictates that electrons fill orbitals from lowest to highest energy, following Hund’s rule (each orbital within a sublevel gets one electron before pairing) and the Pauli exclusion principle (no two electrons can have the same four quantum numbers). Understanding electron configurations is crucial for comprehending an atom’s chemical behavior and its position within the periodic table. A complete electron configuration shows the occupancy of all orbitals, while a condensed configuration utilizes noble gas notation for brevity, focusing on valence electrons, which dictate reactivity.
The Role of the Periodic Table in Determining Electron Configurations
The periodic table provides a powerful tool for predicting electron configurations. The arrangement of elements reflects their electronic structures. The period number (row) corresponds to the principal quantum number (n) of the highest occupied energy level. Elements within the same group (column) share similar valence electron configurations, explaining their recurring chemical properties. For instance, Group 1A (alkali metals) all have one valence electron in an s orbital (ns1), resulting in similar reactivity. Groups 2A (alkaline earth metals) have two valence electrons (ns2). The transition metals (Groups 3B-2B) fill d orbitals, while the lanthanides and actinides fill f orbitals. By understanding the periodic table’s organization, one can readily predict the general electron configuration of an element based solely on its position, simplifying the process and highlighting the connection between structure and properties. This predictive capability is invaluable in studying chemical behavior and trends.
Electron Configuration and Periodic Trends
Electron configurations directly explain several periodic trends. Atomic radius, for example, generally decreases across a period (left to right) due to increasing nuclear charge pulling electrons closer. Conversely, atomic radius increases down a group as additional electron shells are added. Ionization energy, the energy required to remove an electron, generally increases across a period due to stronger nuclear attraction; However, it decreases down a group due to increased shielding and larger atomic size. Electronegativity, the ability of an atom to attract electrons in a bond, also increases across a period and decreases down a group, following similar trends to ionization energy. These trends are not absolute; exceptions exist due to electron-electron repulsions and orbital filling intricacies. Understanding electron configurations provides a fundamental basis for explaining these observed periodic patterns and predicting the behavior of elements based on their position within the periodic table. A visual representation, such as a periodic table with electron configurations, is extremely helpful in understanding these trends.
Predicting Electron Configurations Using the Periodic Table
The periodic table itself serves as a powerful tool for predicting electron configurations. The period number corresponds to the principal quantum number (n), indicating the highest energy level occupied by electrons. Group numbers, particularly for main group elements, provide clues to the number of valence electrons. For example, Group 1A elements (alkali metals) have one valence electron (ns1), while Group 2A (alkaline earth metals) have two (ns2). The filling order follows the Aufbau principle and Hund’s rule, proceeding from lower to higher energy levels. Transition metals (Groups 3B-2B) fill d orbitals, while inner transition metals (lanthanides and actinides) fill f orbitals. By understanding these relationships, one can systematically predict the electron configuration of an element based solely on its position on the periodic table. While exceptions exist, this method provides a robust framework for understanding electron arrangement. A well-designed periodic table PDF with electron configurations visually reinforces this predictive capacity.
Printable Periodic Tables with Electron Configurations⁚ PDFs and Other Formats
Numerous resources offer printable periodic tables incorporating electron configurations. PDF format is particularly convenient for printing and sharing, allowing for customized versions tailored to specific needs. These PDFs often include atomic number, symbol, element name, atomic mass, and the full or condensed electron configuration for each element. Some versions may utilize color-coding to highlight trends or groups, enhancing visual learning. Beyond PDFs, other formats such as PNG images and interactive online tables are also available. The choice of format depends on personal preference and intended use. High-resolution images can serve as visually appealing wall charts or digital wallpapers. Interactive online versions offer the advantage of dynamic exploration, allowing users to access detailed information and visualizations. The availability of multiple formats ensures accessibility for diverse learning styles and technological capabilities.
Utilizing Electron Configurations to Identify Elements
Electron configurations serve as unique “fingerprints” for elements, enabling their identification. Each element possesses a distinct arrangement of electrons in its orbitals, reflecting its atomic structure and properties. By analyzing an element’s electron configuration, its position on the periodic table can be determined. The principal quantum number (n) indicates the period (row), while the filling of orbitals (s, p, d, f) determines the group (column) and the element’s block (s-block, p-block, d-block, or f-block). For instance, an electron configuration ending in ns2np6 signifies a noble gas. Conversely, given an electron configuration, one can pinpoint the element using the periodic table as a reference. This process involves systematically identifying the number of electrons and their distribution across orbitals, matching this information to the corresponding element’s atomic number; This technique proves invaluable in various chemical analyses and problem-solving scenarios.
Applications of Electron Configurations in Chemistry
Electron configurations are fundamental in numerous chemical applications. Understanding electron arrangements helps predict an element’s reactivity and bonding behavior. The valence electrons, those in the outermost shell, are particularly crucial. They determine how an atom interacts with others, forming ionic or covalent bonds. For example, elements with nearly filled valence shells readily gain electrons to achieve a stable configuration, while those with few valence electrons tend to lose them. This explains trends in electronegativity and ionization energy. Electron configurations also predict the formation of ions and the resulting ionic compounds. Furthermore, they are vital in understanding the behavior of transition metals, whose variable oxidation states are directly related to the filling of d-orbitals. Spectral analysis, which identifies elements based on their emission and absorption spectra, heavily relies on the electronic transitions between energy levels, as described by electron configurations. In essence, electron configurations are a cornerstone of modern chemical theory and practice.
Condensed Electron Configurations and Noble Gas Notation
Writing out full electron configurations, especially for heavier elements, can be cumbersome. Condensed electron configurations offer a more efficient shorthand notation. This method leverages the stable electron configurations of noble gases. Noble gases, located in Group 18 of the periodic table, possess completely filled valence shells, making them exceptionally unreactive. In condensed notation, the core electrons are represented by the symbol of the preceding noble gas in brackets, followed by the remaining valence electrons. For instance, the full electron configuration of sodium (Na) is 1s²2s²2p⁶3s¹. The condensed configuration simplifies this to [Ne]3s¹, where [Ne] represents the electron configuration of neon (1s²2s²2p⁶). This significantly reduces the length and complexity of the notation, especially beneficial for elements with many electrons. This approach highlights only the valence electrons, which directly influence the element’s chemical properties and reactivity, making it a powerful tool for understanding chemical behavior and predicting reactions.
Electron Configurations and Chemical Properties
An element’s electron configuration is intrinsically linked to its chemical properties. The arrangement of electrons in an atom’s various energy levels and subshells dictates how it will interact with other atoms. Specifically, the valence electrons—those in the outermost shell—are primarily responsible for an element’s reactivity and bonding behavior. Elements with similar valence electron configurations often exhibit similar chemical properties, a key reason for the periodic table’s organization. For example, elements in Group 1 (alkali metals) all have one valence electron (ns¹), leading to a strong tendency to lose that electron and form +1 ions. Conversely, elements in Group 17 (halogens) have seven valence electrons (ns²np⁵), readily gaining one electron to achieve a stable octet and form -1 ions. This predictable relationship between electron configuration and chemical behavior allows us to understand and predict the types of chemical bonds an element will form (ionic, covalent, metallic) and its overall reactivity. Understanding electron configurations provides a foundational understanding of chemical reactions and compound formation.
Visualizing Electron Configurations⁚ Orbital Diagrams
While electron configurations provide a concise notation of electron arrangements, orbital diagrams offer a more visual representation. These diagrams illustrate the filling of atomic orbitals within subshells, using boxes to represent orbitals and arrows to represent electrons. The Aufbau principle, Hund’s rule, and the Pauli exclusion principle guide the construction of these diagrams. The Aufbau principle dictates that electrons fill orbitals from lowest to highest energy levels. Hund’s rule states that electrons individually occupy each orbital within a subshell before pairing up. The Pauli exclusion principle specifies that each orbital can hold a maximum of two electrons with opposite spins. Therefore, an orbital diagram not only shows the electron configuration but also the spin of each electron. This visual representation helps in understanding concepts like paramagnetism (unpaired electrons) and diamagnetism (all electrons paired). For instance, an orbital diagram for oxygen (1s²2s²2p⁴) would clearly show two unpaired electrons in the 2p subshell, explaining its paramagnetic behavior. Constructing and interpreting orbital diagrams is crucial for understanding atomic structure and chemical bonding.
Electron Configurations and Atomic Size
Atomic size, or atomic radius, exhibits trends across the periodic table directly linked to electron configurations. Moving across a period (left to right), atomic size generally decreases. This is because the increasing nuclear charge pulls the electrons closer to the nucleus despite adding electrons to the same principal energy level. The effective nuclear charge increases, overpowering the slight increase in electron-electron repulsion. Conversely, moving down a group (top to bottom), atomic size increases significantly. This is due to the addition of electron shells, which increases the distance between the valence electrons and the nucleus. The added shielding effect of inner electrons reduces the effective nuclear charge felt by the outermost electrons. Electron configuration helps explain these trends. Elements with similar valence electron configurations within the same group show a more consistent pattern of atomic size changes compared to elements in different groups. Noble gases, for instance, show a gradual increase in size going down their group, while transition metals display a more complex pattern due to the filling of inner d orbitals. Understanding electron configurations provides a fundamental basis for comprehending these periodic trends in atomic size.
Electron Configurations and Ionization Energy
Ionization energy, the energy required to remove an electron from a gaseous atom, is closely tied to electron configuration. Across a period, ionization energy generally increases. This is because the increasing nuclear charge holds the electrons more tightly, making it harder to remove them. The electrons are closer to the nucleus and experience a stronger effective nuclear charge. The added electrons are in the same principal energy level, so the shielding effect is relatively constant. Down a group, ionization energy generally decreases. As you move down, the added electron shells increase the distance between the valence electrons and the nucleus. The increased shielding effect from inner electrons reduces the effective nuclear charge felt by the outermost electrons, making them easier to remove. Exceptions to these trends can arise due to electron configuration specifics. For example, the relatively lower ionization energy of group 13 elements compared to group 12 elements is attributed to the added p electron entering a new, higher energy subshell. Similarly, half-filled and completely filled subshells exhibit greater stability, thus requiring more energy for electron removal. Analyzing electron configurations provides valuable insight into the variations of ionization energy across the periodic table.
Resources for Finding and Using Periodic Tables with Electron Configurations
Numerous online and offline resources offer periodic tables incorporating electron configurations. Many educational websites provide interactive versions, allowing users to explore electron arrangements dynamically. These often include additional information beyond basic electron configurations, such as atomic mass, electronegativity, and other relevant chemical properties. Searching for “periodic table with electron configurations PDF” will yield numerous printable versions in PDF format, suitable for individual study or classroom use. Ensure that the chosen resource accurately reflects current IUPAC recommendations for element names, symbols, and atomic masses. Textbooks dedicated to chemistry and general science frequently include detailed periodic tables with electron configurations in their appendices. Additionally, specialized chemistry handbooks provide comprehensive tables with detailed electron shell information. When utilizing these resources, remember to carefully consider the context of the provided data, paying attention to any notations or abbreviations used to represent electron shell configurations. Always prioritize reputable sources to ensure the accuracy and reliability of the information.